Electronic Metamaterials & Devices Group
Manipulating Ferroelectric Materials
Towards Sustainable Microelectronics
About Us
Suraj is currently a Principal Investigator in MIT’s Research Laboratory for Electronics (RLE).
Goals
from a microelectronics perspective, leveraging collective electronic phenomena, engineered at the unit cell level.
Towards making an impact on technology, our research focuses on manipulating novel-yet-simple CMOS materials towards lab-to-fab translation of electronic devices that demonstrate unprecedented performance.
More Moore
Energy demand from computing has been increasing much faster than the world’s energy production; at this rate, in 20 years, computing will require more electricity than the world can generate; novel energy-efficient computing paradigms are required
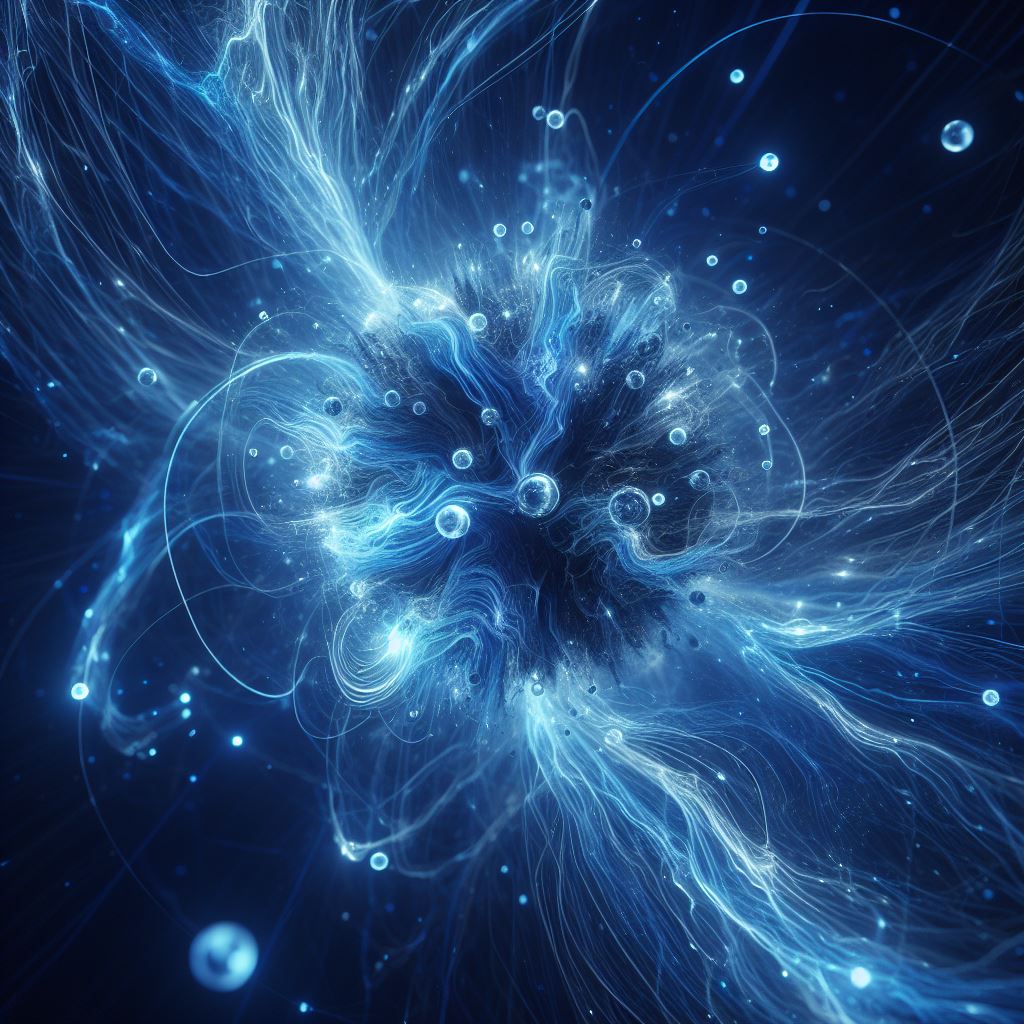
energy-efficient electronics
exploiting collective electronic phenomena
More than Moore
The exponential rise of IoT smart devices (approaching 1 trillion) and energy/heat dissipation in modern microchips demand innovations in self-powered nanotechnologies – spanning rechargeable energy storage, energy harvesting, & power delivery – integrated on-chip
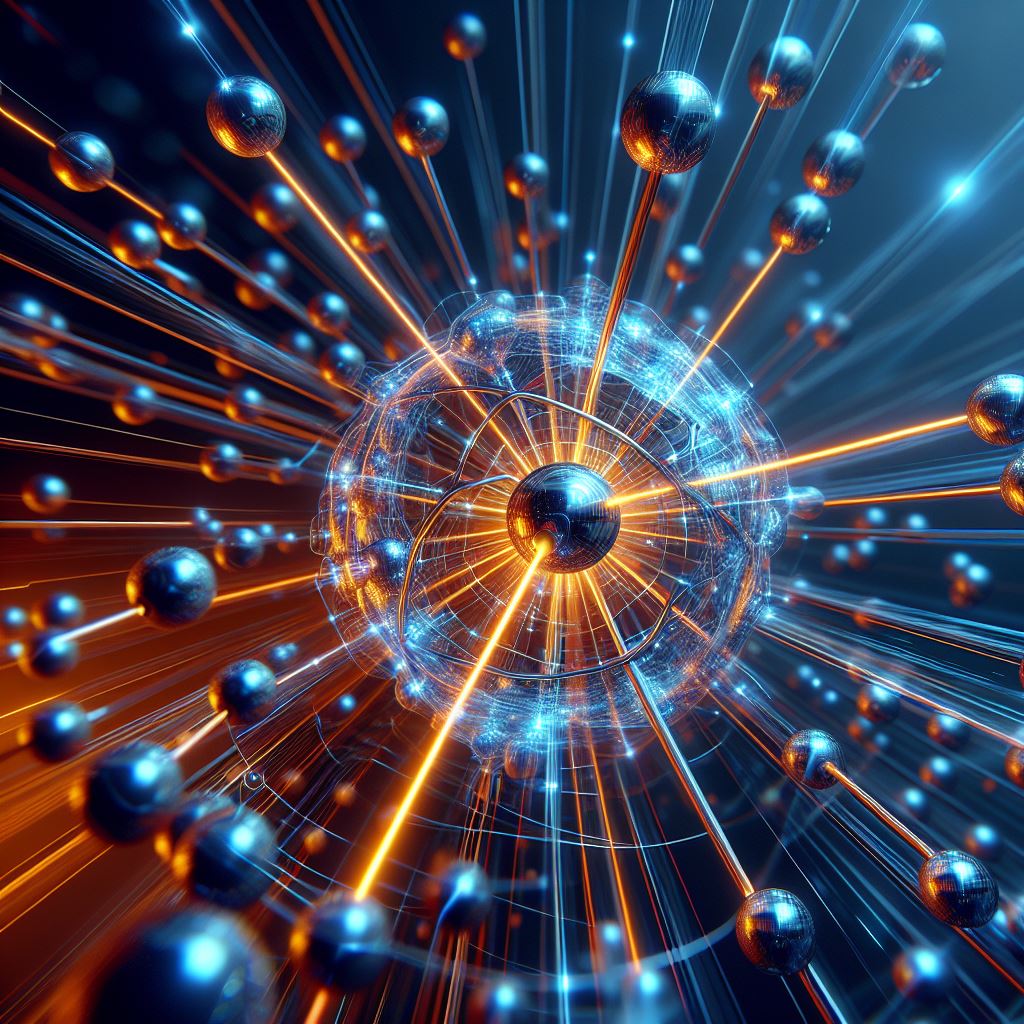
energy-autonomous electronics
ubiquitous self-powered smart devices
Sustainable Microelectronics
3D integration of energy-efficient electronics (computing, memory, AI hardware), energy technologies (energy storage + harvesting, thermal management, power delivery) & sensors on-chip for autonomous data processing i.e. from Cloud to Edge Intelligence
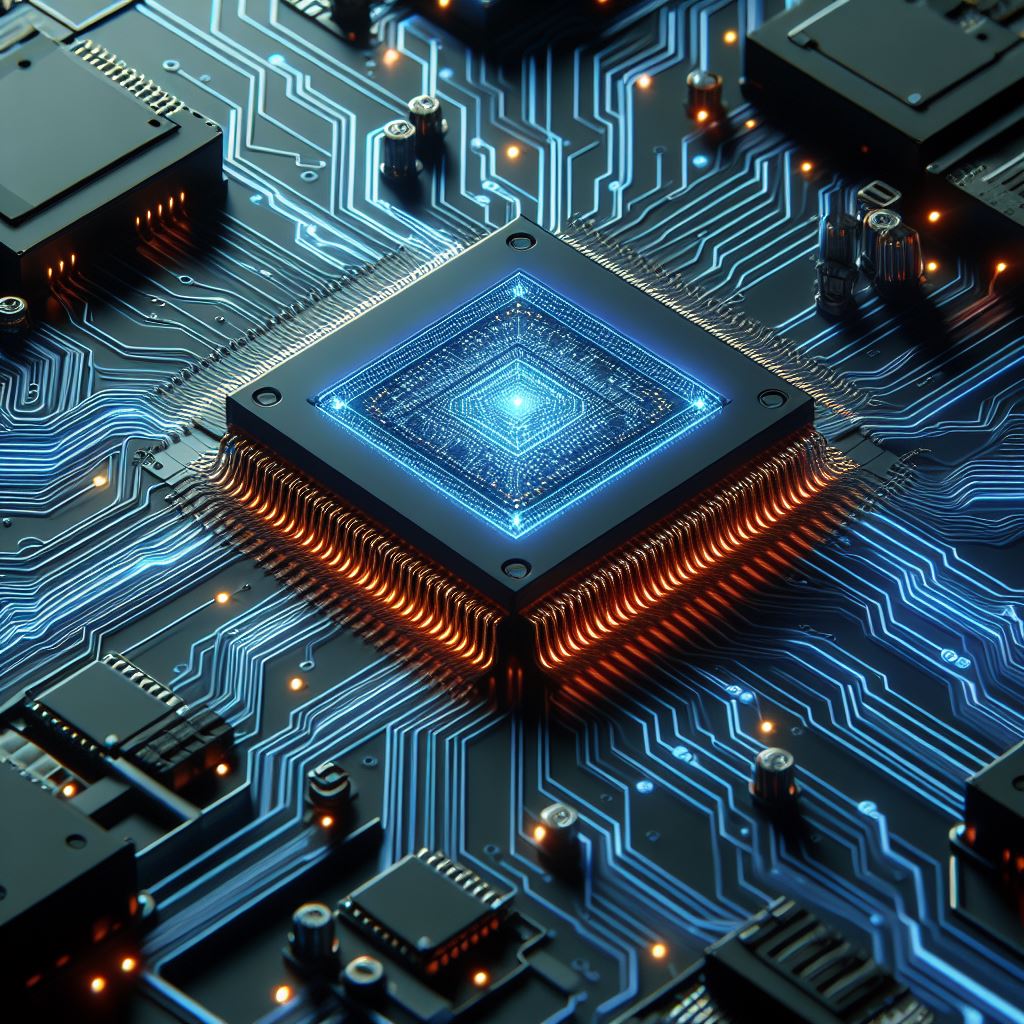
edge electronics
3D-integrated compute+storage+energy+sensing micro-AI-engines
Approach
Materials Science
atomic-scale engineering
Inversion symmetry breaking & phase transitions
Atomic-layer thin films, superlattices, metastable polymorphs
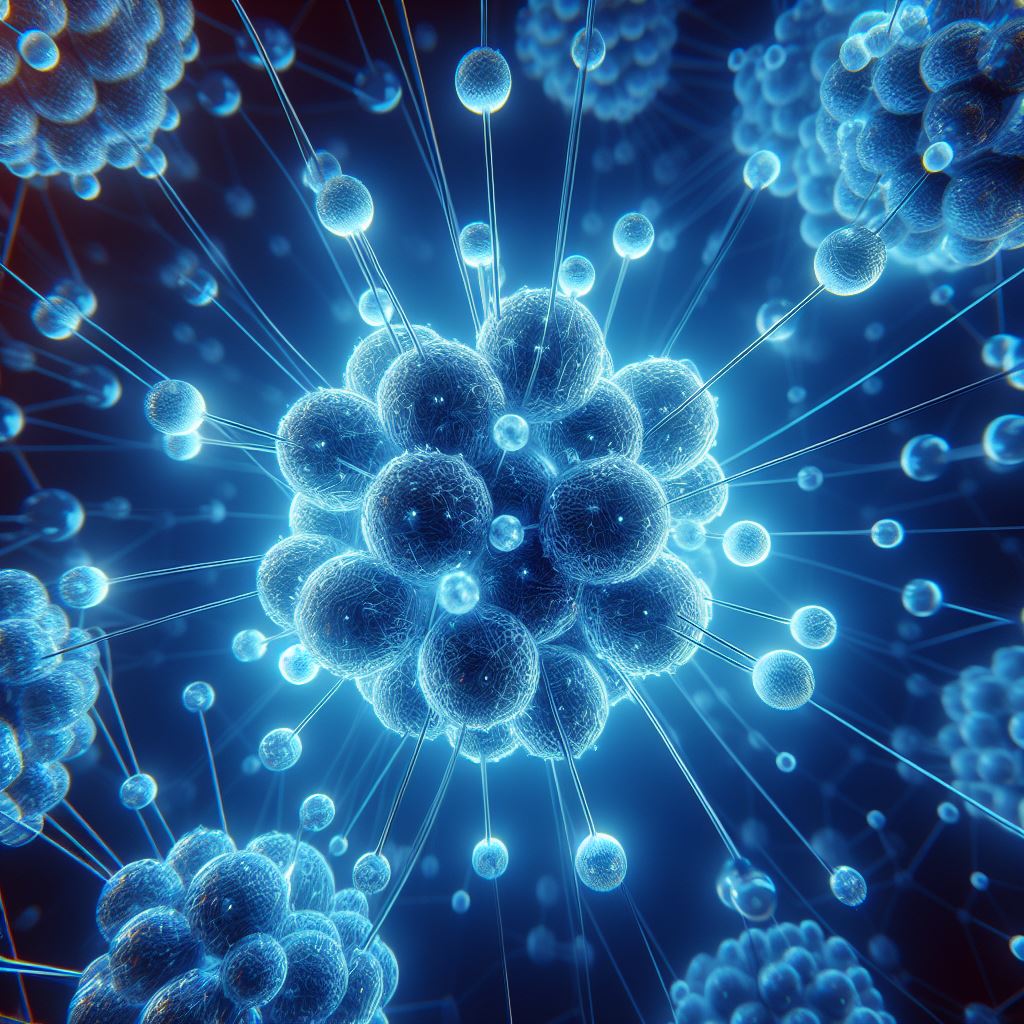
Condensed Matter
emergent (negative) electronic phenomena
Building blocks: collective electronic order, phase transitions
Negative responses: capacitance, piezoelectricity, compressibility
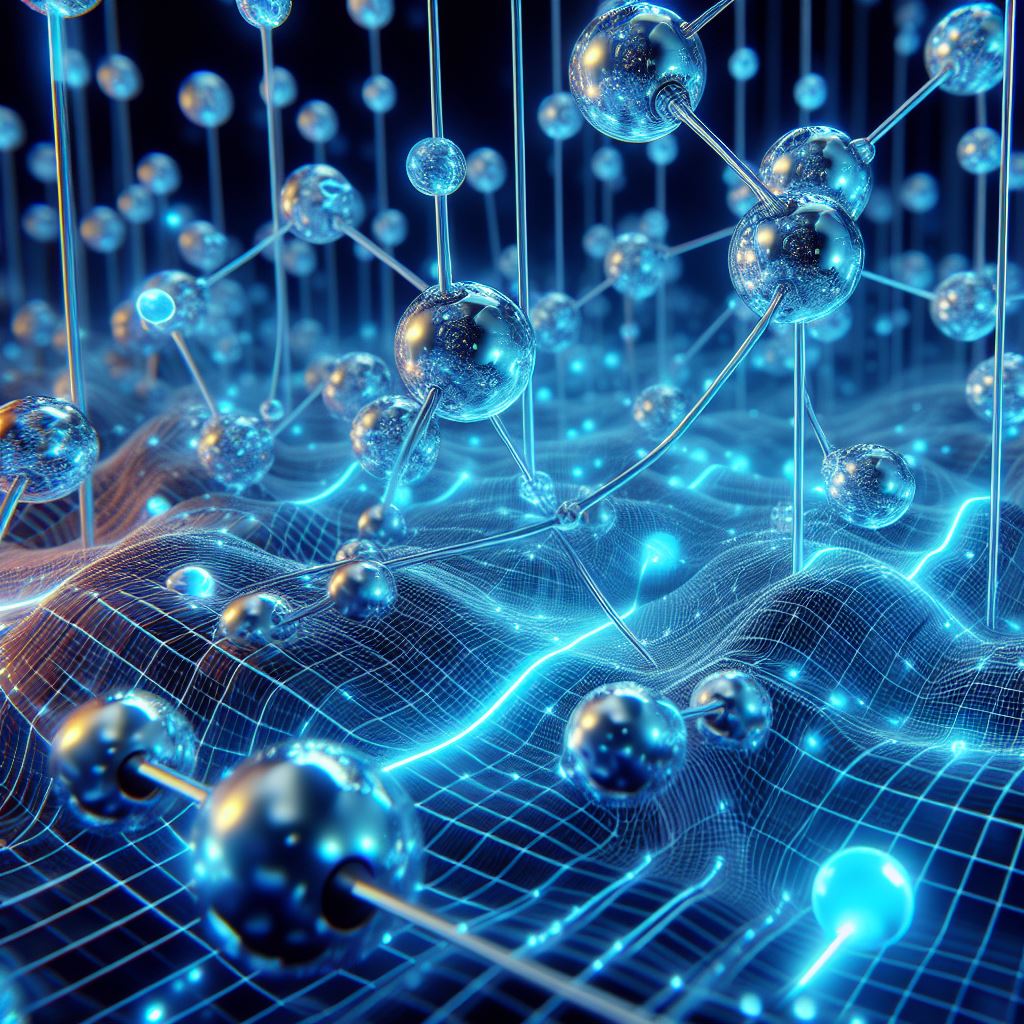
Nanoelectronics
on-chip computing & energy technologies
Computing: logic transistors, nonvolatile memory, AI hardware
Energy: energy storage & harvesting capacitors, power delivery
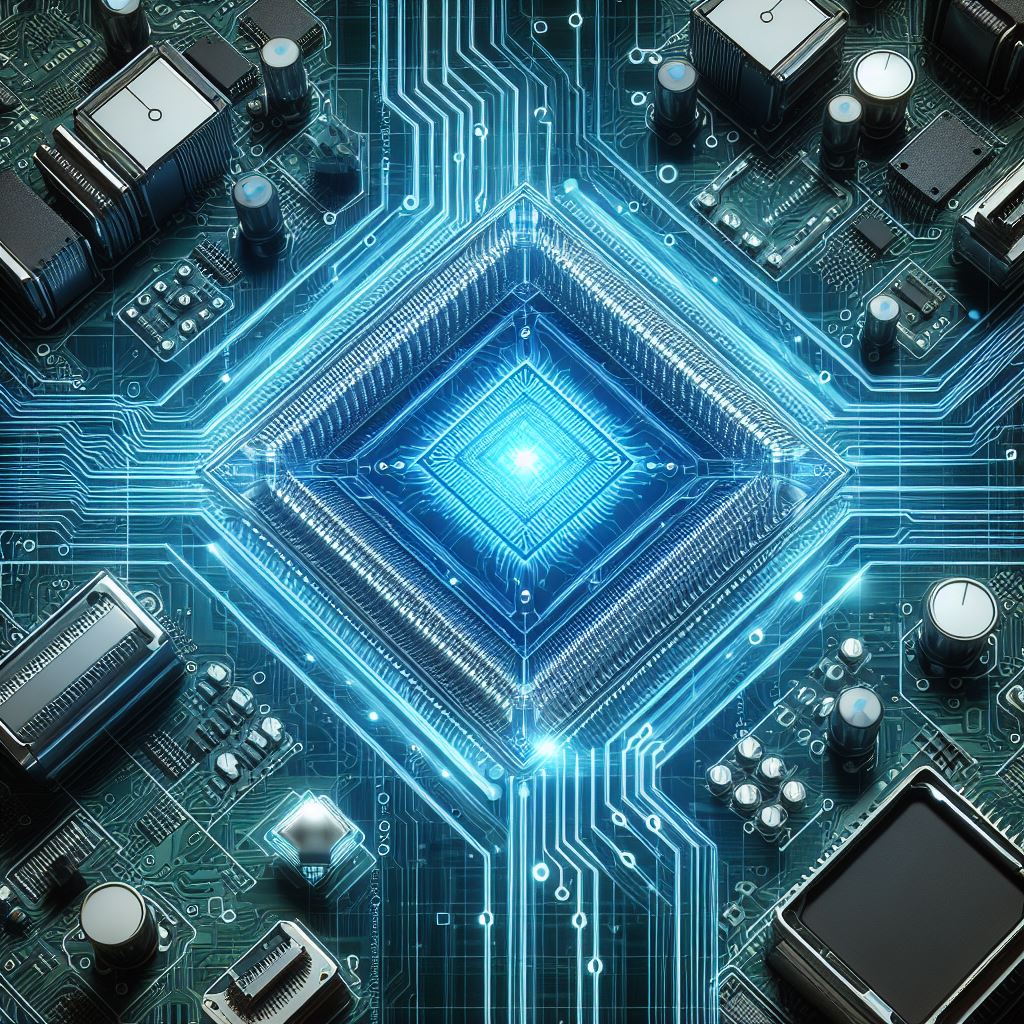
Research Highlights
Harnessing FerroElectronics at the Atomic Scale
Re-imagining electronic materials
Engineering emergent collective electronic order and negative electronic phenomena in otherwise ordinary dielectrics
Cheema et al Nature 2020 | Cheema et al Science 2022
Lab-to-Fab
Samsung Advanced Institute of Technology (SAIT) confirmation of ultrathin ferroelectricity in HfO2-ZrO2 on Si
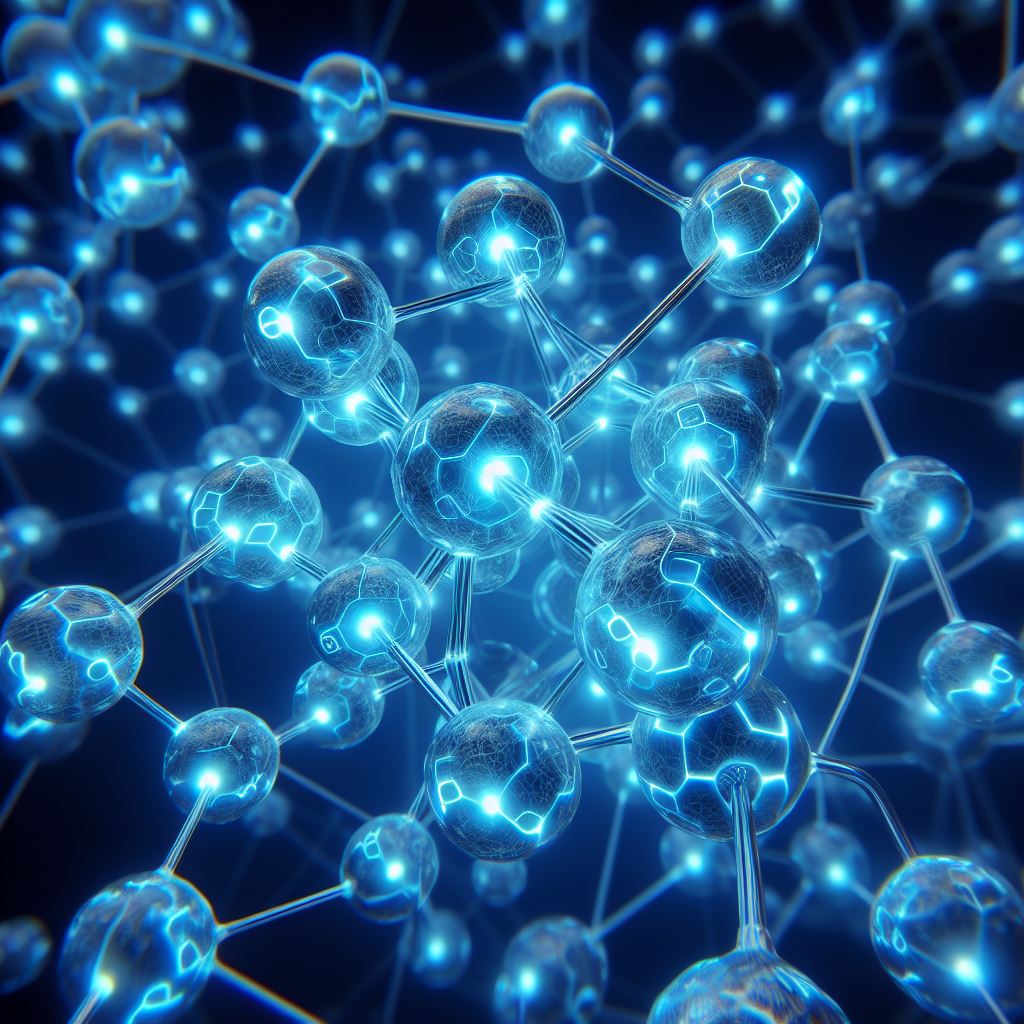
Re-imaginging the transistor
From high-k dielectrics to negative-k ferroelectrics for ultra-low power transistor operation
Cheema et al Nature 2022
Lab-to-Fab
U.S. R&D Foundry confirmation & integration of my NC gate stack into their Defense Foundry transistor technology: IEDM 2022
Samsung Electronics and SAIT confirmation & integration of my NC gate stack into their FinFET technology: Nature Electronics 2023
Intel highlighted my NC technology as a future for energy-efficient computing in their 75th anniversary of the transistor: Science 2022
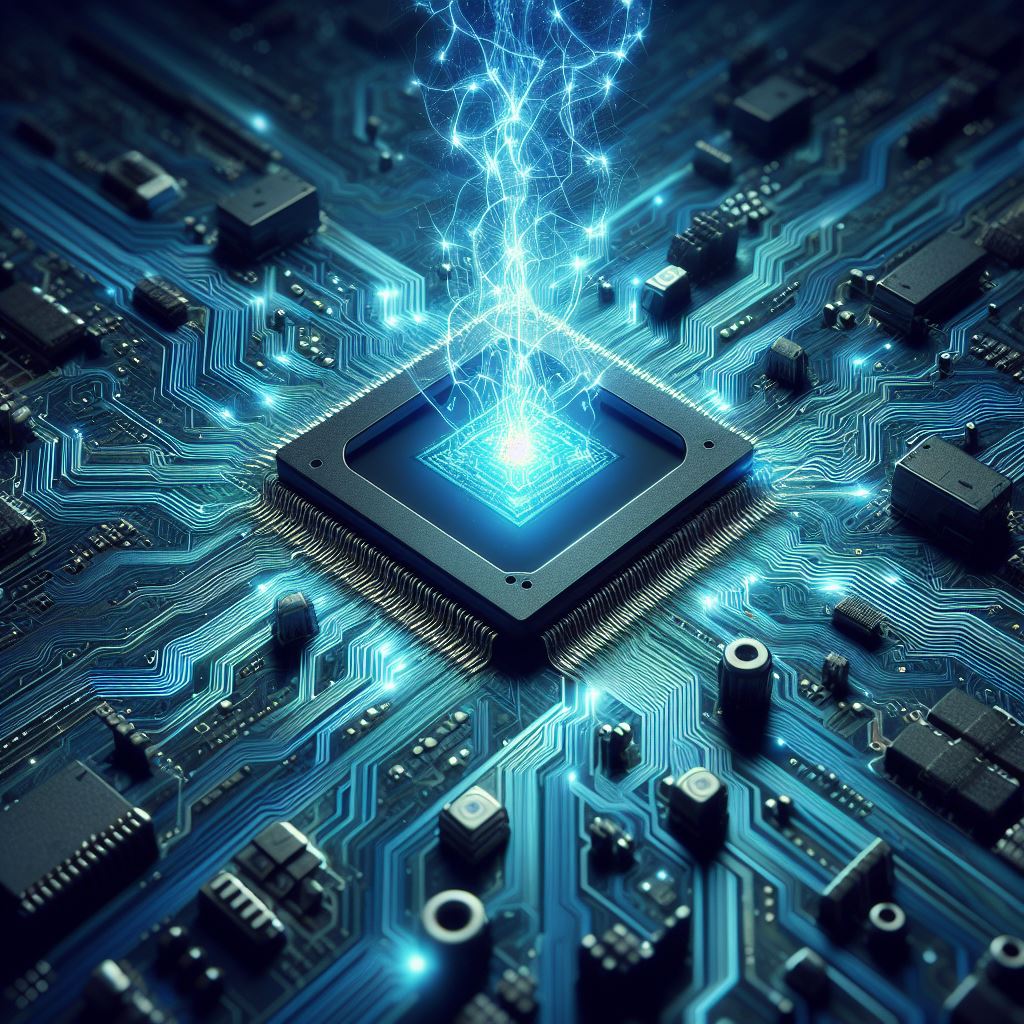
Re-imagining the battery
From electrochemical to electrostatic energy storage for ultrahigh density and ultrafast charging capacitors
Cheema et al Nature 2024
Lab-to-Fab
U.S. R&D Foundry confirmation & integration of my NC energy storage stack into their 3D trench capacitor process: Nature 2024
The Pentagon invitation to present this energy storage technology to US military decision-makers at the Pentagon DARPA Demo Day 2023
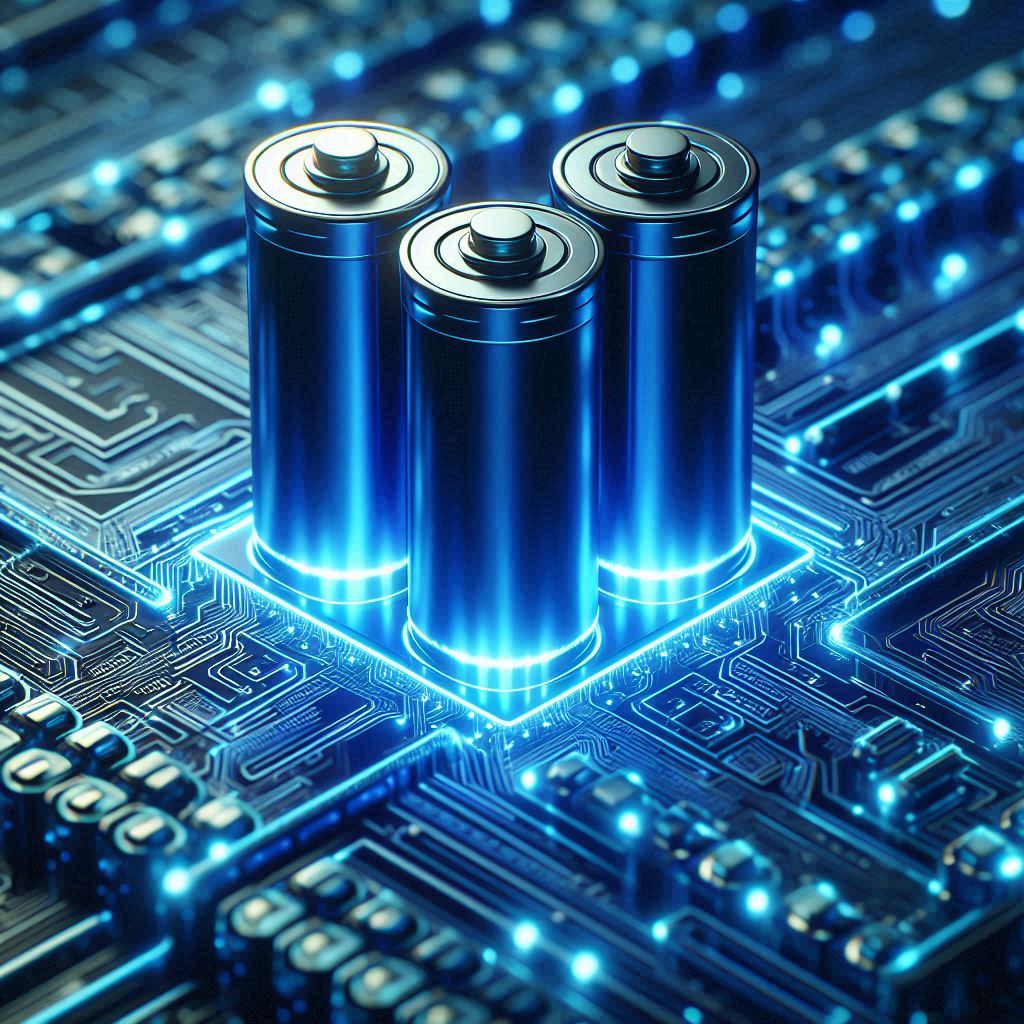
Research Areas
New Paradigms for Electronic Materials & Devices
Materials Design
Computing & Memory
Toolbox
Atoms to Devices
Materials-by-Design Synthesis
To stabilize emergent phenomena beyond the standard unit cell, we utilize Atomic Layer Deposition (ALD) to manufacture hierarchical “super-cells”. ALD, used in today’s microelectronics, deposits atomically-precise films across large-area substrates to enable large-scale integration and facilitate Lab-to-Fab translation.
Thin Film Characterization
To understand the microscopic origins underlying electronic metamaterials, we employ (i) synchrotron x-rays (diffraction, spectroscopy, microscopy), (ii) microscopy (electron, scanning probe), and (iii) transport (ultrafast, cryogenic, etc) at National Laboratories, MIT facilities, and in-house setups.
Electronic Devices
To realize enhanced performance derived from emergent symmetry-broken phenomena, we integrate electronic metamaterials into relevant device structures (e.g. capacitors, transistors) fabricated (i) in-house at MIT.nano (ii) next-door at MIT Lincoln Laboratory and (iii) in collaboration with semiconductor industries.